T cells of the adaptive immune system are not the only cytotoxic cells in the immune system. Natural killer cells (NK cells) are a cytotoxic white blood cell (lymphocyte) of the innate immune system. Clinical trials with NK cells use these as cell-based biologic therapies: The NK cells are the therapy. New research suggests that other types of therapies targeting the proteins that control NK cell function may be useful for treating cancer and that combining NK cell-targeted therapies with immune checkpoint inhibitors may achieve improved responses and reduce toxicity.
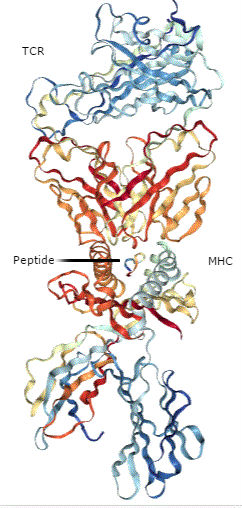
Whereas cytotoxic T cells are activated by a receptor that recognizes peptides that function as antigens when bound to the major histocompatibility complex (MHC) proteins (a process called antigen presentation), NK cells are activated by the NK receptor NKG2D. The NKG2D ligands are proteins of the MICA and MICB (MICA/B) family and the ULBP family, which is also called the RAET1 family (1). Although structurally similar to MHC proteins (Figure 1), these NKG2D ligands do not bind and present peptides. The MICA/B family are transmembrane proteins, and ULBPs can be associated with the outer surface of a cell through a lipid anchor or can have a transmembrane domain. When present on the surface of a target cell, proteins of the MICA/B and ULBP families stimulate a cytotoxic response from the NK cells.
Some cancer cells have increased amounts of these NK cell-activating proteins, which should support NK cell-mediated killing of the cancer cells. Additionally, because the presence of NKG2D ligands indicates a stressed or damaged cell, cancer treatments (especially cytotoxic therapy such as chemotherapy and radiation) can increase the amount of NKG2D ligands on cancer cells. However, cancer cells can release the extracellular parts of these proteins producing soluble forms, which can impair NK cell-mediated killing by binding the receptor NKG2D on the NK cells and blocking the cell-associated ligands from activating the receptor. Furthermore, the genes encoding the NKG2D ligands are highly polymorphic, that is there are many naturally occurring genetic variants, which could contribute to differential effectiveness of NK cell-based or NK cell-targeted treatments.
Zuo and colleagues (2) examined the functional difference between the proteins encoded by the two most common genetic variations in ULBP6, referred to as ULBP0601 or RAETL*01 and ULBP0602 or RAETL*02). ULBP0601 encodes ULBP6 with Arg106Ile147 and ULBP0602 encodes ULBP6 with Leu106Thr147. The variant ULBP0602 is associated with better survival following hematopoietic stem cell transplant, such as would be used for some forms of blood cancer. The authors found that the ULBP6[Leu106Thr147] (Figure 2) bound much more tightly to the receptor NKG2D than did ULBP6[Arg106Ile147] or other NKG2D ligands.
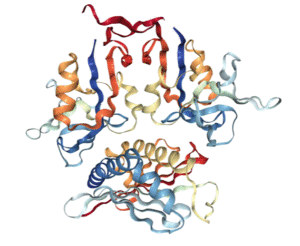
Surprisingly, the higher affinity binding resulted in less NK cell activation and less NK cell-mediated cytotoxicity. Soluble forms of either ULBP6 protein inhibited NK cell-mediated cytotoxicity, but the stronger binding form ULBP6[Leu106Thr147] was the stronger inhibitor. Thus, the allele that produced a protein with less NK-mediated activity and cytotoxicity correlates with better survival in patients receiving stem cell transplant treatment. How this difference in function will affect the outcomes of other NK cell-targeted or NK2GD-targeted cancer therapies remains to be determined.
The receptor NKG2D is present on both NK cells and T cells, on which it also has a stimulating role. Strategies that leverage NKG2D are in preclinical development. Godbersen and colleagues (3) developed a bispecific T cell engager (BiTE), an engineered antibody-like protein with the extracellular part of human NKG2D fused to part of an antibody protein that binds to the T cell receptor. This BiTE is designed to promote the interaction between cytotoxic T cells and tumor cells that have NKG2D ligands. Adding this BiTE to cocultures of T cells and melanoma tumor cells produced a cytotoxic tumor cell response. How these two common ULBP6 variants affect the T cell response is unknown. However, knowing which variant is present in patients may be important to identify the patients that are likely to have the best response to treatments that involve the ULBP6-NKG2D interaction.
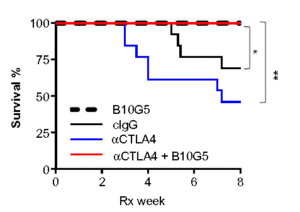
In another preclinical study with mouse cancer models, Zhang and colleagues (4) studied the effect of the soluble form of MICA/B (sMIC) on the response to treatments targeting the CTLA-4 immune checkpoint. In animals with high amounts of sMIC in their circulation because the tumor cells released this molecule from the cell surface, anti-CTLA-4 treatment resulted in larger tumors and more metastasis to the lungs than in animals receiving a control antibody. Furthermore, the animals with high circulating sMIC prior to receiving either the anti-CTLA-4 or control treatment developed colitis and died. Mice receiving combined treatment with an antibody against CTLA-4 and an antibody against sMIC (B10G5) cleared the lungs of metastatic tumors, reduced the size of the primary prostate tumor, and prevented the development of colitis. These animals receiving the combined treatment survived for the duration of the study, regardless of whether circulating sMIC was high prior to treatment or not (Figure 3).
This preclinical study may explain the gastrointestinal immune toxicity observed in some patients receiving immune checkpoint inhibitor therapy targeting CTLA-4 and suggests that testing for sMIC in patients will help identify those at highest risk for this complication.
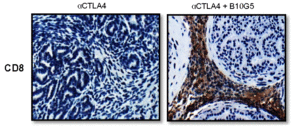
The combined sMIC-targeted and CTLA-4-targeted therapy altered many aspects of the animals’ immune response to the tumor. The mice had increased the number of CD8+ T cells in the spleen, in tumor-draining lymph nodes, and in the tumors (Figure 4). These CD8+ T cells also had increased amounts of a T cell receptor protein, exhibited increased activity, and maintained their ability to be activated (did not become “tolerant”). Combined therapy also affected T cell-stimulating dendritic cells by increasing the amount of the stimulating ligands on tumor-associated dendritic cells. Given that NKG2D is present on both NK cells and T cells, it is likely that effects on both populations of cells contributed to the beneficial response of neutralizing sMIC.
The role of sMIC has been difficult to assess in preclinical animal studies, because mice do not have MIC genes. Thus, Zhang and colleagues had to create a specially engineered mouse cancer model to perform this study. Although the rodent and human immune systems are similar, they have key differences that make not only translating mouse studies to patients, but even developing an appropriate mouse model, difficult. Indeed, the mouse NKG2D ligand MULT-1 triggers tumor cell rejection in mice (5). No human NKG2D ligand with a similar function has been identified. Thus, investigating NKG2D signaling pathways on NK and T cells represents a challenge in using mouse models for studying human disease.
Related Reading
- R. Carapito, I. Aouadi, W. Ilia, S. Bahram, Natural killer group 2, member D/NKG2D ligands in hematopoietic cell transplantation. Front. Immunol. 8, 368 (2017). DOI: 10.3389/fimmu.2017.00368 PubMed
- J. Zuo, C. R. Willcox, F. Mohammed, M. Davey, S. Hunter, K. Khan, A. Antoun, P. Katakia, J. Croudace, C. Inman, H. Parry, D. Briggs, R. Malladi, B. E. Willcox, P. Moss, A disease-linked ULBP6polymorphism inhibits NKG2D-mediated target cell killing by enhancing the stability of NKG2D ligand binding. Sci. Signal.10, eaai8904 (2017). PubMed
- C. Godbersen, T. A. Coupet, A. M. Huehls, T. Zhang, M. B. Battles, J. L. Fisher, M. S. Ernstoff, C. L. Sentman, NKG2D ligand targeted Bispecific T Cell Engagers lead to robust antitumor activity against diverse human tumors. Mol. Cancer Ther. (12 May 2017) DOI: 10.1158/1535-7163.MCT-16-0846 PubMed
- J. Zhang, D. Liu, G. Li, K. F. Staveley-O’Carroll, J. N. Graff, Z. Li, J. D. Wu, Antibody mediated neutralization of soluble MIC significantly enhances CTLA4 blockade therapy. Sci. Adv. 3, e1602133 (2017). PubMed
- J. F. Foley, New connections: Manipulating NK cell responses. Sci. Signal. 10, eaan8369 (2017). PubMed
Cite as: N. R. Gough, Natural killer cells in cancer immunotherapy. BioSerendipity (5 June 2017) https://www.bioserendipity.com/2017/06/05/natural-killer-cells-in-cancer-immunotherapy/ .